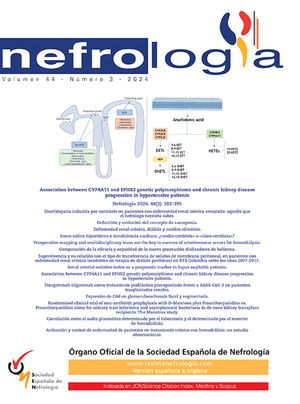
Acute kidney injury (AKI) is a common devastating complication characterized by an abrupt loss of renal function. It is of great significance to explore promising biomarkers for AKI treatment.
Materials and methodsHere, we established LPS (lipopolysaccharide)-induced AKI mice models and LPS-induced AKI mouse renal tubular epithelial cell model. The severity of AKI was determined by the levels of BUN (blood urea nitrogen) and SCr (serum creatinine), the observation of pathological section as well as the renal tubular injury score. The apoptosis was determined by the measurement of Caspase-3 and Caspase-9 activities, and cell apoptosis assays. qRT-PCR (quantitative real-time PCR) and western blot revealed that miR-322-5p (microRNA-322-5p) was up-regulated in LPS -induced AKI models while Tbx21 (T-box transcription factor 21) was down-regulated in LPS-induced AKI models. Dual-luciferase reporter and RNA pulldown assays detected the interaction of Tbx21 with miR-322-5p.
ResultsWe found that miR-322-5p was overtly over-expressed in the in vitro LPS-induced AKI model and promoted the apoptosis of AKI mouse renal tubular epithelial cells via inhibiting Tbx21, which suppressed the mitochondrial fission and cell apoptosis through MAPK/ERK (mitogen-activated protein kinase/extracellular signal-related kinase) pathway.
ConclusionsWe demonstrated that miR-322-5p promotes LPS-induced mouse AKI by regulating Tbx21/MAPK/ERK axis, which might provide new sights for AKI research.
La insuficiencia renal aguda (IRA) es una complicación común devastadora caracterizada por una pérdida abrupta de la función renal. Es de vital importancia explorar biomarcadores prometedores para el tratamiento de la IRA.
Materiales y métodosEstablecimos aquí un modelo de ratones con IRA inducido por lipopolisacáridos (LPS) y un modelo de células epiteliales del túbulo renal en ratones. La severidad de IRA fue determinada por los niveles de NUS (nitrógeno ureico en sangre) y SCr (creatinina sérica), la observación de la sección patológica, así como la puntuación del daño renal tubular. La apoptosis fue determinada mediante la medición de las actividades de Caspasa-3 y Caspasa-9, y los ensayos de apoptosis celular. Las pruebas qRT-PCR (PCR cuantitativa en tiempo real) y Western blot revelaron que miR-322-5p (microRNA-322-5p) se incrementaba en los modelos de IRA inducidos por LPS, mientras que Tbx21 (factor 21 de transcripción de T-box) disminuía en los modelos de IRA inducidos por LPS. Los ensayos del reportador de luciferasa dual y de precipitación pulldown de ARN detectaron la interacción entre Tbx21 y miR-322-5p.
ResultadosEncontramos que miR-322-5p se hallaba manifiestamente incrementado en el modelo in vitro de IRA inducido por LPS, y promovió la apoptosis de células epiteliales del túbulo renal de IRA en ratones mediante la inhibición de Tbx21, lo cual suprimió la fisión mitocondrial y la apoptosis a través de la vía MAPK/ERK (proteína quinasa activada por mitógeno/quinasa relacionada con la señal extracelular).
ConclusionesDemostramos que miR-322-5p promueve la IRA inducida por LPS en ratones mediante la regulación del eje Tbx21/MAPK/ERK, lo cual podría aportar una percepción nueva para la investigación sobre la IRA.
Acute kidney injury (AKI) is a common complication, which is defined by a repaid loss of kidney function based on an increase in the levels of BUN (blood urea nitrogen) and SCr (serum creatinine).1 This life-threatening complication results in two million deaths per year and may be caused by various clinical conditions.2 The categories of AKIs conventionally include prerenal, intrarenal and postrenal causes.3 There are no specific therapies which can attenuate AKI and promote recovery.4 Renal replacement therapy and correcting reversible causes are main options of AKI treatment at present.3 New diagnostic techniques (e.g., renal biomarkers) have the potential of assisting early diagnosis,3 thus it is imperative to explore the potential biomarkers and investigate the underlying mechanism of AKI progression.
MiRNAs (microRNAs) are a class of small non-coding RNAs (ncRNAs) that can act as regulators of their target genes to involve in biological processes.5 MiRNA dysregulation contributes to the development and progression of multiple diseases, including AKI.6 Increasing evidence has proven the potential of miRNAs to act as new diagnostic biomarkers of AKI,7 such as miR-16,8 miR-4949 and miR-101.10 Herein, we investigated the role of potential AKI-related miRNA in AKI. MiRNAs exerts their functions in human diseases usually through direct regulation of their downstream mRNAs (messenger RNAs).11–13 In our current study, as miRNA–mRNA axis in AKI remains largely unknown, we also explored its downstream mRNA and its relevant regulatory mechanism in AKI.
The purpose of this research is to uncover potential AKI-related miRNA and the downstream mechanism. To obtain this aim, we established LPS (lipopolysaccharide)-induced AKI mice model and LPS-induced AKI mouse renal tubular epithelial cell model for the follow-up AKI-focused investigations. Then, we utilized the bioinformatics tool GEO (Gene Expression Omnibus) to screen out miR-322-5p and applied starBase to acquire its downstream target Tbx21, and then explored the impact of miR-322-5p/Tbx21 axis on cell apoptosis in LPS-induced AKI models. Given that MAPK/ERK (mitogen-activated protein kinase/extracellular signal-related kinase) pathway has been shown to be linked to cell apoptosis in multiple cancers,14,15 we hypothesized this pathway was potentially involved in miR-322-5p/Tbx21 axis-regulated LPS-induced AKI.
Materials and methodsCell cultureMouse primary proximal renal tubular epithelial cells were procured from Cell Biologics, Inc. Following the manufacturer's manual, DMEM/F-12 (Dulbecco's Modified Eagle Medium/Nutrient Mixture F-12, Gibco-BRL, Carlsbad, CA, USA) was applied for cell culture, with the addition of 10% FBS (fetal bovine serum; Gibco-BRL). HEK-293T cells supplied by National Institute for the Control of Pharmaceutical and Biological Products (NICPBP) were cultured in DMEM (Gibco-BRL), supplemented with the medium supplements including 10% FBS and 1% Penicillin-Streptomycin Solution (Cat#: 15140122, Gibco-BRL).
LPS-induced AKI mouse models in vivo and in vitroA total of nine 5-week-old mice (female) supplied by Model Animal Institute of Nanjing University were treated with a standard laboratory diet. Animal experiments were approved by the Ethics Committee of the Nanjing First Hospital. LPS was dissolved into normal saline and used for intraperitoneal injection at 10mg/kg for different time.16 The kidney tissue and serum samples were collected at 0, 12 and 24h. Here, LPS-induced AKI mice model was established after treatment with LPS for 24h. To establish in vitro AKI cell model, we treated mouse primary renal tubular epithelial cells with LPS (0, 2.5, 5μg/mL) for different time.17 Here, the mostly used in vitro AKI cell model was constructed with LPS treatment (5μg/mL) for 24h.
Renal function testRenal function was evaluated by measuring the levels of BUN and SCr in LPS-induced AKI mice in line with the described protocol.18 The former was detected using QuantiChrom™ Urea Assay Kit (BioAssay Systems) and the latter using QuantiChrom™ Creatinine Assay Kit (BioAssay Systems), following the protocols of the supplier.
Assessment of renal oxidative stress levelsTo assess oxidative stress in renal tissues of AKI mice after indicated LPS treatment, the levels of oxidative stress marker MDA (malondialdehyde) and antioxidant enzymes including CAT (catalase), SOD (superoxide dismutase) and GSH-Px (glutathione peroxidase) were detected via utilizing commercially available assay kits as per the supplier's directions. MDA level was detected utilizing assay kit supplied by Beijing Xinshengyuan Biomedical Technology Co., LTD. (Beijing, China); SOD and GSH-Px levels were detected by Invitrogen assay kit; CAT level was detected by Thermo Scientific assay kit (Rockford, IL, USA). The experiments were guided by the described protocol.18
Quantitative real-time PCR (qRT-PCR)Total RNA was extracted from mouse primary proximal renal tubular epithelial cells and kidney tissue samples by using Trizol reagent (Takara, Japan). Reverse transcription and cDNA (complementary DNA) synthesis were implemented by using Hifair® III 1st Strand cDNA Synthesis SuperMix for qPCR (Cat#: 11141ES10, Takara, Japan) or miScript II RT Kit (Cat#: 218160, Qiagen, Hilden, Germany). qPCR was performed using qRT-PCR Kit (Cat#: QR0100-1KT, Sigma–Aldrich, St. Louis, MO, USA). Lastly, we calculated relative RNA levels as per the 2−ΔΔCt method.19
Vector construction and cell transfectionShort hairpin RNAs (shRNAs) directly against Tbx21 (sh-Tbx21-1, sh-Tbx21-2, sh-Tbx21-3) and negative control of shRNA (sh-NC), pcDNA3.1 empty vector, and pcDNA3.1-Tbx21 were supplied by GenePharma (Shanghai, China). Cells were placed on the 6-well plates at a density of 7×105 cells per well and incubated for 6h followed by transfection with indicated plasmids using Lipofectamine 2000 (Cat#: 11668019, Invitrogen). MiR-322-5p mimics, miR-322-5p inhibitors, as well as their negative controls (mimic-NC and inhibitor-NC) were constructed by RiboBio (Guangzhou, China), and the sequences are listed in Table 1. Then, 250pmol of miR mimics/inhibitors were transfected into cells incubated in a 6-well plate via Lipofectamine 2000 for 48h.
Western blotWestern blot was carried out as previously described.20 Total proteins were isolated using Total Protein Extraction Kit (Cat#: PROTTOT-1KT, Sigma–Aldrich) in line with the manufacturer's recommendations. In this assay, protein concentration was measured by Bradford Protein Assay Kit (Cat#: P0006C, Beyotime Biotechnology, Shanghai, China). The protein extracts were loaded onto SDS-PAGE (Cat#: P0670-250mL, Beyotime Biotech, Shanghai, China), followed by protein transfer onto PVDF membranes (Sigma–Aldrich). The membranes were sealed with non-fat milk in TBST for 1h at room temperature and subsequently incubated with primary antibodies at 4°C overnight. After TBST washing, the membranes were subjected to 1h of incubation with secondary antibodies, followed by TBST washing. Then, the membranes were incubated with ECL reagent (Absin, Shanghai, China) at room temperature and photographed. The antibodies used in this assay included Anti-β-actin (Cat#: ab8227, Abcam, Cambridge, Mass, USA), Anti-ERK1+ERK2 (phospho T202+Y204; Cat#: ab223500, Abcam), and Anti-T-bet/Tbx21 (Cat#: ab91109, Abcam). β-Actin was used as an internal loading control. For each antibody, the experiment using each set of samples was performed in triplicate.
Measurement of Caspase-3 and Caspase-9 activityThe experiments were conducted as described.21 According to the supplier's recommendations, the activity of Caspase-3 or Caspase-9 in cell lysates was detected using Caspase-3 Colorimetric Assay Kit (KeyGEN Biotech, Nanjing, China) or Caspase-9 Colorimetric Assay Kit (KeyGEN Biotech), respectively.
Dual luciferase reporter assayDual luciferase reporter assay was performed as previously described.22 The pmirGLO vectors were purchased from Promega (Madison, WI, USA). Utilizing Lipofectamine 2000, luciferase reporter vectors (pmirGLO, pmirGLO-Tbx21 3′UTR-WT or pmirGLO-Tbx21 3′UTR-Mut) were co-transfected with 5pmol of NC mimic or miR-322-5p mimic (20μM) into cells. 48h later, relative luciferase activities were detected with Dual-Luciferase® Reporter Assay System (Promega). The sequences for Tbx21 3′UTR are listed in Table 2.
The specific sequence of the 3′UTR region used in the luciferase experiments.
Tbx 3′U TR-WT | GAAAATGCCGCTGAATTGGAAGGTGCCCACTAACTTAGAAAACAGACGCGGG GCTGAGAGCCCCGAGCTCTTCCCCATCCCTTCCCTGTATAGTGATTGGTTGGAG AGGAAGCGGGGCAAGAAGGATTCTGGGGTTTACTTCTTGTTTCCTGGCCCACA AGGAAA TACGACAGGAGTGTCCCCTGCCCCTTTCTCTGCCCGAACTACAGTCA CGA.CCTGGTGCTGCTTCTGACCCCATGGTTCCATGGAGAACGGAGAATGGAC TCCAGAGAGTTTTGGACCCAGAGGGACTTCATGGCTTTCTGCGAGGTGGAGGG GTCGGGGTGGGGAGTCCAGGAGAGCTGCTCTCTTCCCCTGTCCAGTCAGTAAC TTTCAACTGTTGGTCTGACACCTGTGTTAATCTCTGACCTGAAAGTGAAGATA CACGCATTTTTACAACAGCCAGCCAAACAGAGAAGACTCAGGTGACTGCGGG CGGACTGGGCCACCTGCGAGGAGACAAGAGAGGGTGGGTGCAGAGGAAGGGT TTGAAGGGTGCACATTTCACCAGGCGAGGTCACTTTGAACCGGTGTGTACACA CACGGGTGTCTCIIIIIIATTTCTTCGGGAGGGGGGAGGCTATTTATTGTAGAG AGTGGTGTCTGGATGTATTTCTTCTGTTTTGCATCACTTTCTGGAAATAAACAT GGACCTGGTAAA |
Tbx 3′U TR-Mut | GAAAATGCCGCTGAATTGGAAGGTGCCCACTAACTTAGAAAACAGACGCGGG GCTGAGAGCCCCGAGCTCTTCCCCATCCCTTCCCTGTATAGTGATTGGTTGGAG AGGAAGCGGGGCAAGAAGGATTCTGGGGTTTACTTCTTGTTTCCTGGCCCACA AGGAAA TACGACAGGAGTGTCCCCTGCCCCTTTCTCTGCCCGAACTACAGTGT CGTTGCACGACGACGATCTGACCCCATGGTTCCATGGAGAACGGAGAATGGA CTCCAGAGAGTTTTGGACCCAGAGGGACTTCATGGCTTTCTGCGAGGTGGAGG GGTCGGGGTGGGGAGTCCAGGAGAGCTGCTCTCTTCCCCTGTCCAGTCAGTAA CTTTCAACTGTTGGTCTGACACCTGTGTTAATCTCTGACCTGAAAGTGAAGAT ACACGCATTTTTACAACAGCCAGCCAAACAGAGAAGACTCAGGTGACTGCGG GCGGACTGGGCCACCTGCGAGGAGACAAGAGAGGGTGGGTGCAGAGGAAGGG TTTGAAGGGTGCACATTTCACCAGGCGAGGTCACTTTGAACCGGTGTGTACAC ACACGGGTGTCTcllllll ATTTCTTCGGGAGGGGGGAGGCTATTTATTGTAG GAGTGGTGTCTGGATGTATTTCTTCTGTTTTGCATCACTTTCTGGAAATAAACA TGGACCTGGTAAA |
RNA pulldown assay was implemented as previously described.23 The biotin-labeled probes (including Bio-NC, Bio-Tbx21-WT, and Bio-Tbx21-Mut) was obtained from RiboBio. Later, Cell lysates were mixed with indicated probes overnight, and then subjected to incubation with streptavidin-coated magnetic beads (Sigma–Aldrich) for 2h at 4°C. The biotinylated RNA complexes were pulled down and the enrichment of RNA was measured via qRT-PCR.
Terminal-deoxynucleoitidyl transferase mediated nick end labeling (TUNEL)TUNEL assay was conducted for the evaluation of cell apoptosis as previously described.24 The transfected cells underwent 15min of fixation in 4% paraformaldehyde at room temperature. In situ Cell Death Detection Kit (Roche, Mannheim, Germany) was used to perform TUNEL staining as per the supplier's manual. Lastly, TUNEL-positive cells were counted under a fluorescence microscope (Cat#: XSP-63B, Shanghai Optical Instrument Factory No.1).
Flow cytometry apoptotic analysisFlow cytometry analysis was implemented to test cell apoptosis as previously described.25 After incubation, the cells were collected and dyed by Annexin V-FITC/PI from BD Biosciences (San Diego, CA, USA), followed by analysis with BD Flow Cytometer (BD Biosciences, Franklin Lakes, NJ, USA) analysis.
PAS (Periodic Acid-Schiff) stainingPAS staining was implemented as previously described.26 The section of LPS-treated mice's kidney tissue samples was stained using PAS (Cat#: Ab150680, Abcam). Pathological section was reviewed under a confocal microscope (Smartzoom 5, Zeiss, Shanghai, China).
Histological analysisThe renal tubular injury was characterized with deformation of vacuoles, loss of brush border, kidney tubular dilation or nuclear condensation. The severity of tubular injury was scored as per the following scoring system: 0 to 4 based on the percentage of the injured area of the section (0=no change; 1=changes affecting<25%; 2=changes affecting 25–50%; 3=changes affecting 50–75%; 4=changes affecting>75% of the section). At least six different visual fields were randomly chosen under a confocal microscope (magnification, ×400; Smartzoom 5, Zeiss) and the average score was calculated.
Observation of mitochondrial morphologyMitochondrial morphology was detected with the help of Mitotracker Red CMXRos (Invitrogen) as per the previous protocol.27 Mitochondrial branch length was observed by the inverted microscope (Nikon Ti-E, Nikon Corporation) and the mean branch length was measured by ImageJ.
U0126 treatmentU0126 served as the inhibitor of MAPK/ERK signaling pathway.28 U0126 (Cat#: HY-12031, Med Chem Express, Monmouth Junction, NJ, USA) treatment was performed as per the manual of the supplier.
Statistical analysisBio-repeats in each experiment were implemented in triplicate and technical replicates were repeated three times for each bio-repeat. Data analysis was processed with the application of SPSS 22.0 (SPSS Inc., Chicago, IL, USA). The experimental data were presented as mean±SD (standard deviation). In this study, group difference was analyzed by Student's t-test, one-way ANOVA (analysis of variance) or two-way ANOVA, followed by Dunnett or Tukey correction. P value less than 0.05 indicated data were statistically significant.
ResultsIn this research, we established LPS-induced AKI in vitro model and in vivo model for the following investigations to explore the underlying mechanism of AKI. Bioinformatics tools and experiments were utilized to determine the object of research (miRNA of interest: miR-322-5p) and its downstream target (Tbx21). We then performed TUNEL staining and detected the activity of apoptotic markers (Caspase-3 and Caspase-9) to evaluate the impact of miR-322-5p on cell apoptosis in LPS-induced AKI model. Given that stimulation of MAPK/ERK signaling pathway is linked to cell apoptosis, we further probed into whether it was involved in the process of miR-322-5p/Tbx21 axis affecting the apoptosis of in vitro AKI model.
Establishment of LPS-induced AKI mice model and mouse renal tubular epithelial cell modelGiven that the levels of BUN and SCr represent the severity of AKI, we firstly measured the levels of BUN and SCr in LPS-treated mice. The results showed that both serum BUN and SCr levels in LPS-treated mice markedly were elevated as the time of LPS treatment went by (Fig. 1A, B; *P<0.05, **P<0.01). Then, we observed swollen renal tubular epithelial cells, deformed vacuoles, and abnormally narrowed renal tubules in the pathological section of LPS-treated mice's kidney tissues and found that the symptoms were getting worse as time went by (Fig. 1C), which suggested the increasing severity of AKI. LPS treatment exacerbated renal tubular injury in LPS-treated mice (Fig. 1D; **P<0.01). Consistent with this, we also observed that the level of oxidative stress in AKI mice renal was increased with LPS treatment time, since LPS increased the level of MDA and reduced the activities of SOD, CAT and GSH-Px in a time-dependent manner (Fig. S1A–D; **P<0.01). In addition, we assessed the activity of apoptotic markers, including Caspase-3 and Caspase-9. The results demonstrated that both Caspase-3 activity and Caspase-9 activity in renal tubular cells of LPS-treated mice were significantly enhanced as the time of LPS treatment (Fig. 1E, F; *P<0.05, **P<0.01). Meanwhile, we also detected that the levels of caspase-3, caspase-9 and Bax were elevated whereas the level of Bcl-2 was reduced in renal tissues from AKI mice along with the increasing LPS treatment dose (Fig. S1E). Moreover, we observed that TUNEL-positive cells in LPS-treated mice's kidney tissues were greatly increased as well (Fig. 1G, H; **P<0.01). Together, we confirmed that LPS-induced AKI mice model was established successfully. Given that the highest efficiency of 24-h LPS treatment, we chose it for in vivo experiments in this research.
Establishment of LPS-induced AKI mice model and mouse renal tubular epithelial cell model. For LPS-induced AKI mice model, mice were intraperitoneally injected with LPS (10mg/kg) for 0, 12, and 24h. (A, B) The BUN and SCr levels were examined via qRT-PCR analysis (one-way ANOVA, Tukey). (C) The images of pathological section of LPS-treated mouse kidney tissue samples stained by PAS were shown (scale bar: upper: 20μm, lower: 5μm). Red arrows indicate swelling of renal tubular epithelial cells; black arrows indicate tubular lumen shrinkage; white arrows indicate vacuolar deformation. (D) The LPS-treated mice model's renal tubular injury score was marked ranging from 0 to 4: 0 (no change); 1 (changes affecting<25%); 2 (changes affecting 25–50%); 3 (changes affecting 50–75%); 4 (changes affecting>75%) (one-way ANOVA, Tukey). (E, F) The activity of Caspase-3 and Caspase-9 of renal tubular cell in LPS-induced AKI mice model was shown (one-way ANOVA, Tukey). (G) The apoptosis of LPS-treated mice's kidney tissue section was determined by TUNEL assay. (H) The TUNEL-positive cells were measured in the LPS-treated mouse renal tubular epithelial cell model at different time points (one-way ANOVA, Tukey). (I) For LPS-treated mouse renal tubular epithelial cell model, cells were treated with LPS (5μg/mL) for 0, 12, and 24h. The apoptosis rate of in vitro LPS-induced AKI model was increased as time went by (one-way ANOVA, Tukey). (J, K) Caspase-3 and Caspase-9 activities were determined in LPS-treated mouse renal tubular epithelial cell model (one-way ANOVA, Tukey). **P<0.01, *P<0.05.
Next, we measured the apoptosis rate of LPS-treated mouse renal tubular epithelial cells. The apoptosis rate showed an obvious increase in a time-dependent manner (Fig. 1I; **P<0.01). Meanwhile, Caspase-3 and Caspase-9 activities were enhanced by LPS treatment (Fig. 1J, K; *P<0.05, **P<0.01). Thereby, we confirmed the successful establishment of in vitro AKI cell model. Since 24h of LPS treatment presented the higher efficiency than 12-h LPS treatment, we chose it for in vitro experiments in this research.
miR-322-5p promotes the apoptosis of AKI renal tubular epithelial cellsWe next explored a functional miRNA in AKI. With the application of GSE130796 (screening condition: log2 | FC |>1.0, Padj<0.05), we obtained five putative miRNAs (miR-122-5p, miR-126a-5p, miR-322-5p, miR-200a-5p, and miR-374-5p). Then qRT-PCR detected that both miR-126a-5p and miR-322-5p were elevated by LPS treatment at 24h in AKI renal tubular epithelial cells (Fig. 2A, **P<0.01). Further, we explored whether the expression of miR-126a-5p and miR-322-5p was affected by LPS in a dose- or time-dependent manner. As illustrated in Fig. 2B (*P<0.05, **P<0.01), the expression level of miR-322-5p, instead of miR-126a-5p, was heightened with the treating time of LPS in AKI mice renal tissues. Likewise, the results in AKI cell model were consistent with those in in vivo model (Fig. 2C, D; *P<0.05, **P<0.01). Therefore, we speculated that miR-322-5p might be able to regulate the apoptosis of AKI renal tubular epithelial cells.
miR-322-5p promotes the apoptosis of AKI renal tubular epithelial cells. (A) Relative expression levels of putative miRNAs in LPS-treated mouse renal tubular epithelial cell model (5μg/mL; 24h) were detected via qRT-PCR (Student's t-test). (B) The expression levels of miR-126a-5p and miR-322-5p in renal tubular cells of AKI mice models were measured via qRT-PCR in mice under LPS treatment for different time (one-way ANOVA, Tukey). (C, D) The expression levels of miR-126a-5p and miR-322-5p were measured in mouse renal tubular epithelial cells under LPS treatment at the same dose of 5μg/mL for different time or with different doses for 24h (one-way ANOVA, Tukey). (E, F) The apoptosis rate was increased by miR-322-5p mimic and decreased by miR-322-5p inhibitor (Student's t-test). (G, H) Caspase-3 activity was promoted by miR-322-5p and was inhibited by miR-322-5p inhibitor (Student's t-test). (I, J) Caspase-9 activity was enhanced by miR-322-5p and was repressed by miR-322-5p inhibitor (Student's t-test). The experiments as shown in (E–J) were performed in LPS-induced AKI mouse renal tubular epithelial cell model (5μg/mL; 24h). **P<0.01, *P<0.05.
We then evaluated the regulatory role of miR-322-5p on the apoptosis of AKI renal tubular epithelial cells. The effect of miR-322-5p overexpression or deficiency on the apoptosis rate of AKI renal tubular epithelial cells was identified by flow cytometry apoptotic analysis. The results displayed that up-regulation of miR-322-5p increased the apoptosis rate while knockdown of miR-322-5p exhibited the opposite effect (Fig. 2E, F; **P<0.01), indicating that miR-322-5p promotes the apoptosis of AKI renal tubular epithelial cells. Additionally, we measured the impact of miR-322-5p on the activity of apoptotic markers. The results showed that elevation of miR-322-5p enhanced caspase-3 activity while inhibition of miR-322-5p decreased caspase-3 activity (Fig. 2G, H; **P<0.01). Also, the caspase-9 activity was promoted by elevation of miR-322-5p but suppressed by ablation of miR-322-5p (Fig. 2I, J; **P<0.01). Taken together, miR-322-5p accelerates the apoptosis of AKI renal tubular epithelial cells.
miR-322-5p promotes the apoptosis of AKI renal tubular epithelial cells via inhibiting Tbx21Moreover, we probed into the molecular mechanism of miR-322-5p in AKI renal tubular epithelial cells. Previous literatures have reported that miRNAs play a role in regulating target gene expression.29 Through the preliminary prediction on the starBase website (http://starbase.sysu.edu.cn/), we selected six putative mRNAs associated with cell proliferation, morphology, and apoptosis in cancers, including Grb2,30 Rictor,31 Tbx21,32 Ddx3x,33 Ube4b,34 and Vegfa35 (Fig. 3A). Subsequently, we measured the expression levels of above candidate mRNAs in renal tubular cells of LPS-induced AKI mice model (10mg/kg) and in LPS-induced AKI renal tubular epithelial cell model (5μg/mL). The results showed that Tbx21 was significantly down-regulated in both LPS-induced AKI in vivo and in vitro models (Fig. 3B; **P<0.01). Tbx21 were thereby selected for the subsequent investigations. To explore the effect of miR-322-5p on Tbx21 expression, we measured the mRNA level and protein level of Tbx21 under miR-322-5p mimic/inhibitor treatment. Tbx21 levels were visibly decreased by up-regulation of miR-322-5p (Fig. 3C, D; **P<0.01), whereas those of Tbx21 were obviously increased by miR-322-5p silencing (Fig. 3E, F; **P<0.01), indicating that miR-322-5p negatively modulates Tbx21 expression.
miR-322-5p promotes the apoptosis of AKI renal tubular epithelial cells by targeting Tbx21. (A) Six candidate downstream mRNAs of miR-322-5p were screened out by starBase (http://starbase.sysu.edu.cn/). (B) The expression levels of six mRNAs were detected in renal tubular cells of LPS-induced AKI mice model (10mg/kg; 24h) and LPS-induced AKI mouse renal tubular epithelial cell model (5μg/mL; 24h) (Student's t-test). (C) qRT-PCR tested that Tbx21 mRNA level was reduced under miR-322-5p mimic treatment (Student's t-test). (D) Western blot revealed that the protein level of Tbx21 was lowered under miR-322-5p mimic treatment (Student's t-test). (E, F) Tbx21 mRNA and protein level under miR-322-5p inhibition were tested by qRT-PCR and western blot, respectively. Student's t-test. (G, H) The knockdown efficiency and overexpression efficiency of Tbx21 were verified by qRT-PCR analysis (one-way ANOVA, Tukey; Student's t-test). (I) The apoptosis rate was increased by Tbx21 knockdown (one-way ANOVA, Dunnett). (J) The apoptosis rate was decreased by Tbx21 overexpression (Student's t-test). (K, L) Caspase-3 activity was measured responding to Tbx21 knockdown (one-way ANOVA, Dunnett) and Tbx21 up-regulation (Student's t-test). (M, N) Caspase-9 activity was measured in cells with Tbx21 knockdown (one-way ANOVA, Dunnett) or overexpression (Student's t-test). (O) The luciferase activity was promoted by miR-322-5p overexpression in pmirGLO-Tbx21 3′UTR-WT group (two-way ANOVA, Tukey). (P) miR-322-5p was relatively enriched in Bio-Tbx21-WT group (one-way ANOVA, Tukey). (Q–S) Tbx21 deficiency could weaken the repressive effect of miR-322-5p inhibition on the apoptosis via measuring the apoptosis rate, Caspase-3 and Caspase-9 activities, respectively (one-way ANOVA, Tukey). The experiments as shown in C–S were performed in LPS-induced AKI mouse renal tubular epithelial cell model (5μg/mL; 24h). **P<0.01, *P<0.05.
Besides, we investigated the effect of Tbx21 on the apoptosis of AKI renal tubular epithelial cells. The interference efficiency and overexpression efficiency of Tbx21 were elucidated by qRT-PCR analysis. In view of the higher knockdown efficiency of sh-Tbx21-1 and sh-Tbx21-2 plasmids (Fig. 3G; **P<0.01), we chose sh-Tbx21-1 and sh-Tbx21-2 for the following experiments; overexpression of Tbx21 was confirmed as qRT-PCR detected a significant increase in Tbx21 level in transfected cells with pcDNA3.1-Tbx21 (Fig. 3H; **P<0.01). Through apoptosis assays, we found that depletion of Tbx21 significantly enhanced the apoptosis rate of LPS-induced AKI renal tubular epithelial cell model while overexpression of Tbx21 led to the reverse results (Fig. 3I, J; **P<0.01). Likewise, Caspase-3 and Caspase-9 activities were strengthened by Tbx21 silencing but impaired by Tbx21 overexpression (Fig. 3K–N; **P<0.01). Altogether, Tbx21 negatively modulates cell apoptosis in AKI renal tubular epithelial cells.
To further explore the physical interaction of miR-322-5p and Tbx21, we performed luciferase reporter and RNA pulldown assays in LPS-induced AKI mouse renal tubular epithelial cell model. As shown in Fig. 3O (**P<0.01), the luciferase activity of pmirGLO-Tbx21 3′UTR-WT was dramatically inhibited by miR-322-5p mimic while that of pmirGLO-Tbx21 3′UTR-Mut had no obvious change, suggesting that miR-322-5p could bind to Tbx21. The results of RNA pulldown assays also verified the same finding since miR-322-5p was overtly abundant in Bio-Tbx21-WT group instead of mutated group (Fig. 3P; **P<0.01). Rescue experiments were subsequently carried out to determine the impact of miR-322-5p/Tbx21 axis on cell apoptosis in AKI cell model. We found that Tbx21 knockdown attenuated the suppressive effect of downregulated miR-322-5p on the apoptosis rate of AKI renal tubular epithelial cells (Fig. 3Q; **P<0.01). In the meantime, silenced Tbx21 impaired the repression of miR-322-5p knockdown on the Caspase-3 activity and Caspase-9 activity as depicted in Fig. 3R and S (*P<0.05, **P<0.01). In conclusion, miR-322-5p enhances the apoptosis of AKI renal tubular epithelial cells via negatively modulating Tbx21.
Tbx21 modulates mitochondrial fission and cell apoptosis through MAPK/ERK signaling pathwayAs MAPK/ERK pathway has been reported to be associated with cell apoptosis,14,15 we next investigated whether Tbx21 affects the MAPK/ERK signaling pathway. Western blots showed that the protein level of p-ERK1/2 and p-MAPK was increased after 24h of LPS treatment (Fig. 4A; **P<0.01), implying the activation of MAPK/ERK signaling pathway in renal tubular cell of LPS-induced AKI mice model. As shown in Fig. 4B, p-ERK1/2 also showed a higher protein level after 24h of LPS treatment (*P<0.05, **P<0.01), displaying the stimulation of MAPK/ERK signaling pathway in LPS-induced AKI renal tubular epithelial cell model. Later, we explored the impact of Tbx21 on the MAPK/ERK signaling pathway. The results of western blot suggested that Tbx21 deficiency obviously increased p-ERK1/2 protein level (Fig. 4C; *P<0.05, **P<0.01). Simultaneously, overexpression of Tbx21 largely reduced the protein level of p-ERK1/2 (Fig. 4D; **P<0.01). In a word, Tbx21 could impede the stimulation of MAPK/ERK pathway in LPS-induced AKI mice model and cell model.
Tbx21 modulates mitochondrial fission and cell apoptosis through MAPK/ERK signaling pathway. (A, B) The protein level of p-ERK1/2 was increased in renal tubular cell of LPS-induced AKI mice model (10mg/kg; 24h) and LPS-induced AKI mouse renal tubular epithelial cell model (5μg/mL; 24h), individually (Student's t-test). (C, D) The protein level of p-ERK1/2 was increased by Tbx21 knockdown (one-way ANOVA, Dunnett) and was decreased by overexpressed Tbx21 in LPS-induced AKI mouse renal tubular epithelial cell model (5μg/mL; 24h) (Student's t-test). (E) The mitochondria became rounded, small and fragmented in LPS-treated mouse renal tubular epithelial cell model (5μg/mL; 24h). Scale bar=10μm. (F) The mean branch length was reduced in LPS-treated mouse renal tubular epithelial cell model (5μg/mL; 0, 12, 24h) (one-way ANOVA, Tukey). (G) The mitochondria became rounded, small and fragmented after down-regulating Tbx21. Scale bar=10μm. (H) The mean branch length was reduced after Tbx21 knockdown (one-way ANOVA, Tukey). (I) The mitochondria became tabulated after overexpressing Tbx21. Scale bar=10μm. (J) The mean branch length was increased after down-regulation of Tbx21 (Student's t-test). (K–O) U0126 treatment was used to inhibit the activity of MAPK/ERK signaling pathway. The inhibition of this pathway weakened the promoting effect of Tbx21 knockdown on the apoptosis via observing the mitochondrial morphology and measuring the mitochondrial mean branch length, the apoptosis rate, Caspase-3 and Caspase-9 activities, respectively (one-way ANOVA, Tukey). Scale bar=10μm. The experiments as shown in (G–O) were performed in LPS-induced AKI mouse renal tubular epithelial cell model (5μg/mL; 24h). **P<0.01, *P<0.05.
Since the association between mitochondrial fission and cell apoptosis has been reported in previous studies,36,37 we then investigated the effect of Tbx21 on mitochondrial morphology. Before that, we analyzed the mitochondrial morphology in LPS-induced AKI cell model. The mitochondria got rounded, small and fragmented upon LPS treatment (Fig. 4E), suggesting that the mitochondria were injured in LPS-induced AKI cell model. The mean branch length of mitochondria was significantly decreased caused by LPS treatment (Fig. 4F; *P<0.05, **P<0.01), exhibiting the improved degree of mitochondrial fission. Next, we observed the mitochondrial morphology and found that upon ablation of Tbx21, the mitochondria got rounded, small and fragmented and the mean branch length was decreased (Fig. 4G, H; **P<0.01). On the contrary, the mitochondria became tubular and the mean branch length was increased upon up-regulation of Tbx21 (Fig. 4I, J; **P<0.01). The above results verified that Tbx21 suppresses mitochondrial fission in LPS-induced AKI renal tubular epithelial cell model. Afterwards, we used the inhibitor of MAPK/ERK pathway U012628 to further verify whether Tbx21 affects mitochondrial fission through the MAPK/ERK pathway. The mitochondria became tabulated under U0126 treatment compared to sh-Tbx21-1 group (Fig. 4K). The measurement results of the mean branch length also verified the same finding as the mean branch length was relatively increased in sh-Tbx21-1+U0126 group compared to that in sh-Tbx21-1 group (Fig. 4L; *P<0.05, **P<0.01). To sum up, Tbx21 negatively regulates mitochondrial fission via MAPK/ERK signaling pathway.
Moreover, we detected the apoptosis rate of AKI mouse renal tubular epithelial cell model with indicated transfections. The data exhibited that the apoptosis rate was lower in sh-Tbx21-1+U0126 group compared to that in sh-Tbx21-1 group (Fig. 4M; **P<0.01). Additionally, Caspase-3 and Caspase-9 activities were lowered in sh-Tbx21-1+U0126 group compared to that in sh-Tbx21-1 group (Fig. 4N, O; *P<0.05, **P<0.01). Thus, we concluded that Tbx21 negatively modulates mitochondrial fission and cell apoptosis via MAPK/ERK signaling pathway.
DiscussionAKI is a common and serious problem threatening millions per year, resulting in deaths and disability.38 Previous studies have confirmed the correlation between miRNAs and AKI.39 For instance, miR-668 down-regulates MTP18 to preserve mitochondrial dynamics in IAKI (ischemic acute kidney injury).40 miR-214 ameliorates AKI by impairing apoptosis via targeting DKK3 and stimulating the Wnt/β-catenin pathway.41 In our study, we found a novel functional miRNA in AKI. Herein, we identified that miR-322-5p expression was increased in LPS-induced AKI model in time- and dose-dependent manner. More importantly, we found that miR-322-5p promotes the apoptosis of AKI renal tubule epithelial cells.
Besides, we uncovered the regulation mechanism of miR-322-5p in AKI. Given that miRNAs could modulate target gene expression,42 we investigated potential miR-322-5p target in this research. Previous studies have identified several targets of miR-322-5p, such as Smad7,43 IGF-1,44 TSPAN5,45etc. Here, we presented a novel downstream target gene of miR-322-5p, Tbx21. Tbx21 has been proven to be a suppressor of MAPK/ERK signaling pathway in thyroid cancer.46 Stimulation of MAPK/ERK signaling pathway is linked to cell apoptosis. For example, miR-497 promotes apoptosis through negative regulation of RAF-1-mediated MAPK/ERK signaling pathway.47 Based on this, we speculated that miR-322-5p promotes the apoptosis via repressing Tbx21, which might inactivate the MAPK/ERK signaling pathway in AKI. Through the loss-of-function and gain-of-function experiments, we identified the inhibitory effect of Tbx21 on cell apoptosis in AKI models. We also manifested the mechanism by which Tbx21 regulated cell apoptosis in AKI through MAPK/ERK pathway. In this study, we demonstrated that Tbx21 inactivates MAPK/ERK signaling pathway in AKI.
Mitochondria are highly dynamic organelles undergoing regular fission and fusion cycles.48 Mitochondrial fission is related to apoptosis and often occurs in AKI.36 Alterations in mitochondrial morphology are related to changes in metabolism, cell death and cell development.49 In addition, previous studies have highlighted the therapeutic potential of blocking excessive mitochondrial fission in the treatment of human diseases.50 In this research, we found that Tbx21 suppressed the mitochondrial fission and cell apoptosis. Intriguingly, the inhibition of MAPK/ERK signaling pathway weakened the inhibitory effect of Tbx21 on mitochondrial fission and cell apoptosis. Thus, we identified that Tbx21 modulates mitochondrial fission and cell apoptosis through MAPK/ERK signaling pathway.
ConclusionIn conclusion, the present study demonstrated that miR-322-5p enhanced the apoptosis of AKI renal tubular epithelial cells via inhibiting Tbx21 expression. Additionally, we revealed that miR-322-5p activated MAPK/ERK signaling pathway to modulate the apoptosis of AKI mouse renal tubular epithelial cells. Further investigations will focus on other signals modified by miR-322-5p except Tbx21/MAPK/ERK axis in the future research.
Authors’ contributionsLi Fan: conception and design, administrative support.
Xiaodong Liu: collection and assembly of data, data analysis and interpretation.
Xiaobing Ji and Xiangxiang Li: collection and assembly of data, data analysis and interpretation.
Xin Du: collection and assembly of data, data analysis and interpretation.
Manuscript writing: all authors.
Final approval of manuscript: all authors.
Statement of ethicsAnimal study was approved by the Ethics Committee of the Nanjing First Hospital.
Data availability statementNot applicable.
Funding sourcesThis research did not receive any specific grant from funding agencies in the public, commercial, or not-for-profit sectors.
Conflict of interestThe authors have no conflicts of interest to declare.
Thanks for all members.