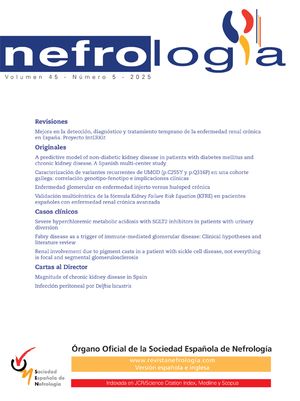
Hemodialysis wastewater contains high concentrations of ammonia nitrogen and phosphorus. Recovery of these nutrients as soil fertilizers represents an interesting opportunity to ensure a sustainable fertilizer supply.
MethodsIn this paper, a simple method for recovering phosphorous and nitrogen as crystalline struvite [MgNH4PO4·6H2O] is presented. An integrated cost model is also presented in order to create a positive business case.
ResultsRecovery rates in form of struvite of 95% of PO43−-P and 23% of NH4+-N were achieved with a profit.
ConclusionTo the best of our knowledge, this paper is the first to study the recovery of these naturally occurring minerals from hemodialysis wastewater. This offers great potential for the valorization of this type of wastewater.
El agua residual de la hemodiálisis contiene altas concentraciones de nitrógeno amoniacal y fósforo. La recuperación de dichos nutrientes como fertilizantes del suelo representa una oportunidad interesante para garantizar un suministro de fertilizantes sostenible.
MétodosEn este documento se presenta un método simple de recuperar fósforo y nitrógeno en forma de cristales de estruvita [MgNH4PO4·6H2O]. También se presenta un modelo de costes integrado para crear un caso de negocio positivo.
ResultadosSe lograron unas tasas de recuperación en forma de estruvita del 95% de PO4 3−-P y el 23% de NH4 +-N, con beneficio.
ConclusiónSegún nuestros conocimientos, este documento es el primero que estudia la recuperación de estos minerales de origen natural del agua residual de la hemodiálisis, lo cual ofrece un gran potencial para la valorización de este tipo de aguas residuales.
Hemodialysis consumes huge volumes of water and produce large quantities of wastewater containing many potential resources like organic matter, nitrogen, and phosphorus.1 Therefore, recovery and reuse of these nutrients as agricultural fertilizers is highly desirable.1,2 We have previously demonstrated that hemodialysis wastewater is a valuable resource that can provide fit-for-purpose water,3 energy,4 nutrients,1,2 and carbon emission savings.5
The discharge of wastewater in hemodialysis is high and could be estimated annually at approximately 98 million cubic meters over the world and 18 million cubic meters in United States.6,7 In our country, Morocco the discharge is estimated at 1 million cubic meters each year.6,7 In a world where demands for freshwater are ever growing, and where limited water resources are increasingly stressed by over-abstraction, pollution and climate change, neglecting the opportunities arising from improved wastewater management is nothing less than unthinkable.5,8,9
Hemodialysis wastewater which contains a high amount of phosphorus and nitrogen would be a good source of struvite (MgNH4PO4·6H2O – magnesium ammonium phosphate hexahydrate).10 This precipitate, due to its chemical characteristics, represents an effective alternative source of rock phosphate fertilizer for vegetables and plants growth.11 Nitrogen and phosphorus in wastewaters are also a burning environmental issue. Recovering these elements from wastewater can also help reducing the amount of phosphorus entering the environment and thus lowering the environmental impact.11
To the best of our knowledge, this paper is the first to study the recovery of this naturally occurring mineral from hemodialysis wastewater. This offers great potential for the valorization of this type of wastewater.
Materials and methodsSampling and analysesWater samples were obtained from a single dialysis facility. Wastewater was collected from the outflow pipe that drains hemodialysis effluent (spent dialysate) directly into the municipal sewage line. Samples were stored at 4°C soon after sampling until used in struvite precipitation tests.
Samples were analyzed for the concentration of cations, anions, chemical oxygen demand (COD), ortho-phosphate (PO43−-P) and total ammonium nitrogen (NH4+-N). The cation concentrations were determined using an ICP-OES, type Perkin Elmer Optima 3000 DV (Waltham, Massachusetts, USA). The anion concentrations were determined using an ion chromatography system, type Metrohm IC Compact 761 (Schiedam, The Netherlands). NH4+-N was analyzed using test kit LCK 303. PO43−-P was analyzed using test kit LCK 348 and COD was analyzed using test kit LCK 314 (all Dr. Lange, HACH, Loveland, Colorado, USA) in a spectrophotometer HACH XION 500 (HACH, Loveland, Colorado, USA). The biological oxygen demand (BOD) was determined using the OxiTop® system (WTW, Germany) over a period of 5 days at 20°C.
Crystallizations experimentsA 5l laboratory-scale, single-cell electrochemical batch reactor was used for struvite production (Fig. 1). The pH and temperature values were monitored through online probes. In all experiments, magnesium chloride hexahydrate (MgCl2·6H2O) was added to meet a molar ratio [Mg2+: Ca2+>2] and [Mg2+: PO43−>1], which were selected based on data reported by Li et al.12 and Liu et al.13 who found struvite crystals with high purity and high phosphorus recovery when using these molar ratios. Crystallization was attained at electric current density of 55Am−2. The obtained struvite cake from the process was dried at room temperature to form a powder.
Struvite precipitate characterizationThe crystalline nature and the semi quantitative composition were determined by X-ray diffraction (XRD; Bruker D2 Phaser), using struvite standard X-ray diffraction patterns. Phosphorus precipitation in the crystallizer was assessed using “The percentage of precipitated phosphorus as struvite” (PMAP, %).
PMAP was calculated as the ratio of the number of precipitated mmoles of struvite (n(MAP)) (mmol), which was assumed to be the lowest value between the precipitated mmoles of Mg2+, PO43−-P and NH4+-N and the precipitated mmoles of PO43−-P in the solution, as shown in the equation below14:PMAP (%)=n (MAP)preciptitated(PO43−-P)preciptitated×100
ResultsCharacterization of hemodialysis wastewaterThe characteristics of the wastewater are shown in Table 1; the average concentration of PO43−-P and NH4+-N was 13.2mgL−1 and 4.2mgL−1 respectively. The samples had an average pH of 7.53. The BOD was found to be approximately 8.13mgL−1, and the COD was 1053mgL−1.
Results of physico-chemical characterization of effluents from hemodialysis. The average was obtained from four different samples obtained on different days.
Composition | Average | Range |
---|---|---|
Temperature (°C) | 23.5 | 22–25 |
pH | 7.53 | 6.91–8.15 |
COD (mgL−1) | 1053 | 986–1120 |
BOD (mgL−1) | 8.13 | 7.98–8.28 |
NH4+-N (mgL−1) | 4.2 | 3.05–5.35 |
PO43−-P (mgL−1) | 13.2 | 12.32–14.08 |
Sodium (mgL−1) | 3289 | 3243.4–3334.6 |
Potassium (mgL−1) | 93.7 | 88–99.4 |
Magnesium (mgL−1) | 52.1 | 49.8–54.4 |
Calcium (mgL−1) | 86.2 | 81.9–90.5 |
Alkalinity (mgL−1 as CaCO3) | 1715 | 1667–1763 |
COD (chemical oxygen demand) is the amount of dissolved oxygen that must be present in water to oxidize chemical organic materials.
BOD (biochemical oxygen demand) is the amount of dissolved oxygen used by microorganisms in the biological process of metabolizing organic matter in water.
Under optimal conditions, the removal efficiencies of PO43−-P and NH4+-N in struvite precipitation were analyzed as approximately 95% and 23%, respectively (Table 2). The phosphorus removal as struvite was similar at the three pH conditions evaluated (i.e., pH 8, 9 and 10). Although, the removal of NH4+-N increased as the pH value increased (Fig. 2). The nitrogen removal can be explained as a combination of the precipitation and volatilization processes, the latter favored under well-mixing conditions at higher pH levels.15 Temperature has a relatively less significant effect on struvite precipitation than the pH level. However, the temperature strongly influences the efficiency of phosphate removal.
Removal efficiency of phosphorus and nitrogen.
T (°C) | 20 | 20 | 20 | 25 | 35 | 40 |
---|---|---|---|---|---|---|
pH | 8 | 9 | 10 | 9 | 9 | 9 |
Removal efficiency (%) | ||||||
PO43−-P | 91.5±0.2 | 92.6±0.1 | 91.9±0.3 | 97.5±0.2 | 98.6±0.0 | 98.2±0.1 |
NH4+-N | 14.6±1.4 | 22.3±0.7 | 40.1±0.4 | 16.3±1.1 | 20.5±0.4 | 27.4±1.2 |
MAP precipitates | ||||||
PMAP (%) | 70.4±0.3 | 83.4±1.6 | 82.8±0.9 | 92.5±0.4 | 77.3±1.8 | 90.1±1.0 |
Mass (g) | 3.9±0.0 | 3.7±0.1 | 4.2±0.1 | 4.4±0.0 | 4.1±0.2 | 4.1±0.1 |
PMAP: the percentage of phosphate as struvite.
The X-ray diffractogram obtained in the analysis of the solids precipitated shows a good correlation between the peaks of the diffractogram obtained for the solid collected in the reactor and the peak of the struvite pattern which confirms that the solid formed was mainly struvite. Phosphorus precipitation in the crystallizer was higher at a pH of 9 and 10 than at a pH of 8, and the mean PMAP was 82.8%.
Economic efficiency of struvite productionWe did an estimate of the financial efficiency of struvite production based on a tailor-designed system for a medium sized dialysis facility working with 20 machines filled to capacity and 2shifts/day and generating approximately 3000l of wastewater (150l of waste dialysate is being generated during each dialysis session: assuming a DFR of 500ml/min, 120L of dialysate is produced during a typical 4H hemodialysis session+30L of water needed for chemical disinfection of the hemodialysis monitor5). The system was designed to produce struvite on a regular basis (2.4kg of struvite can be produced daily). The system consists of wastewater collection tank; a struvite reactor for struvite precipitation and a pilot scale rotating biological contactor (RBC) to treat a small fraction of the effluent from struvite production before disposing it to the environment (Table 3). Data on costs and benefits are shown in Table 4.
Operating parameters for economic evaluation of struvite production from hemodialysis wastewater.
Parameters | Results |
---|---|
Reactor size (l) | 500 |
Cycles per day | 6 |
Struvite recovery efficiency (%) (PO43−-P/NH4+-N) | 90/20 |
Daily struvite production (kg) | 2.44 |
Yearly struvite production (kg) | 760 |
Molar Mg:P ratio | 1.1 |
Yearly required MgSO4 (kg)16 | 45 |
Yearly required MgSO4 evaluation was performed according to the method by Agudosi et al.16
Estimating cost of nutrients recovery as struvite.
Operations parameters | Results |
---|---|
Equipment cost (USD) | 1350 |
Wastewater storage tank: 3m3 (USD) | 180 |
Additional costs (fittings, pipes, etc.) (USD) | 80 |
Estimated total investment (USD) | 1610 |
Required MgSO4 price (USD/kg)17 | 0.33 |
Operations costs (USD/year) | 100.3 |
Maintenance costs (USD/year) | 48 |
Operating duration (year) | 10 |
Struvite market price (USD/kg)18 | 0.8 |
Annual cash inflow (USD) | 608 |
Payback period (month) | 42 |
Recognition that hemodialysis wastewater is an economic resource capable of supplying water,3 nutrients,1,2 energy4 and other valuable materials and services4,5 has become a major driving force to improve effective wastewater management.5 Each year, for instance, approximately 98 million cubic meters of hemodialysis wastewater are generated globally.6,7 Theoretically, the resources embedded in this wastewater would be enough to irrigate and fertilize hundreds of thousands of hectares of crops.
This effluent can also maintain considerable thermal energy quantities, which is discharged to the sewer system with temperature ranging from 20 to 25°C. It is estimated that 1698GWh per year of thermal energy is lost in sewers in dialysis units all over the world and 314GWh in the US (the specific thermal capacity of wastewater is: 1.16kWh/m3×K; the wastewater in the effluent will be cooled down to 5°C, so that 15K can be extracted).19
Globally, hemodialysis wastewater is estimated to contain enough energy to heat 141,500 homes (the average home requires around 12,000kWh of heat/year),20 with an annual fuel cost savings of 118 million Euros if recovered by using technologies like heat exchangers and reused to satisfy heating demands (12,000kWh heat demand/3kW heat production per unit of electricity=4000kWh of electricity. Average residential electricity price in Europe is 0.21 euros).21
Hemodialysis wastewater maintains good amount of carbon, nitrogen and phosphorus, of which have large impacts on the environment. Wastewater from hemodialysis can disrupt aquatic ecosystems with deleterious impacts on aquatic biodiversity, landscapes and recreational opportunities.22 Recovery of nutrients from wastewater can alleviate major environmental problems related to nutrient pollution in ground and surface water sources.21 In this pilot study, recovery rates of 95% of PO43−-P and 23% of NH4+-N were achieved.
Application of struvite in the agricultural sector promises to be a profitable investment. It has been demonstrated that generating 1kg of struvite per day is enough to fertilize 2.6ha of arable land by applying phosphorus (as P2O5) at a rate of 40kg/ha year.20 In this study we were able to demonstrate that an hemodialysis facility with 20 chairs and 2shifts/day could generate approximately 2.4kg of struvite per working day with a profit, and a possibility to fertilize 5.2ha of arable land (estimation based on 6 working days/week). Regarding the environmental cost, the use of wastewater in lieu of synthetic fertilizers can results in an important carbon emissions saving. De Vries et al., have estimated that the use of struvite as fertilizer can results in saving of −0.35kg CO2 eq.kg−1 of struvite.23
ConclusionThe recovery of phosphorus and nitrogen from wastewater is a model for sustainable innovation in hemodialysis. Removing nutrients from where they should not be and using them to create a new generation of enhanced-efficiency fertilizer is the smart thing to do economically and the right thing to do environmentally.
Conflict of interestsThe authors declare they have no conflict of interest.